

This result relies on the adsorptive redistribution of the gas molecules on the graphene surface (equivalent to amplifying the gas concentration), hence facilitating the interaction between ultra-confined graphene plasmons and gas molecules. The rotational-vibrational modes of the gas molecules NO 2, N 2O, NO, and SO 2, which are generally important in environmental and military monitoring applications, as well as in medical diagnostics, are unambiguously detected and identified using the designed graphene nanostructures.
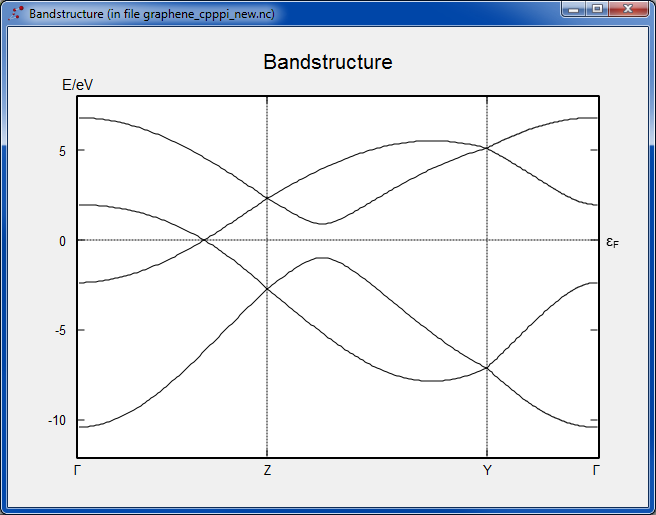
In the present study, we identify gas molecules using graphene plasmons. However, if one could redistribute these gas molecules closer to the graphene surface (e.g., through adsorption, optical forces, or dielectrophoresis forces), it might be possible that the additional enhancement due to plasmonic light confinement can reveal the molecule vibrational modes. Furthermore, the large spatial mismatch between the evanescent plasmon field (~tens of nanometers) and dispersed gas molecules limits the detection region to the immediate surroundings of the graphene layer, thus imposing another serious constraint to the applicability of graphene plasmons to gas sensing.

For example, a 500-μm-thick NO 2 layer with a concentration of 1000 ppm has the same optical density (~0.25%) as an ~10-nm-thick poly(methyl methacrylate) layer 29, 30. However, there is a technologically important challenge to extend solid-sample sensing to gas sensing with SEIRA: 19, 20, 34, 35 the dielectric response of gases at ambient pressure is >4 orders of magnitude weaker than that of solid molecular layers due to the difference in density 18, 19. In particular, ultrasensitive graphene plasmons have been demostrated to be able to detect 0.6 nm thickness of perylene-3,4,9,10-tetracarboxylic dianhydride (PTCDA) and a chemical bond vibration in acetone and hexane vapor 32. Recently, graphene-plasmon-based surface-enhanced infrared absorption (SEIRA) spectroscopy 27, 28, 29, 30, 31, 32, 33, relying on the coupling of molecular vibrational modes with graphene plasmon resonances, has been shown to provide a label-free method to identify trace solid-state molecules, such as protein monolayers 28 and nano-sized polymer films 29, 30. This is mainly due to the fact that the intrinsic detection variations (e.g., differences in electrical conductivity or resonance wavelength) in these devices are not directly correlated with the components and structures of the gas molecules 22, 23, 24, 25, 26, and therefore, these methods are unable to identify molecular species without molecular labels (Supplementary Table 1 and 2). However, the identification of trace gases has been fundamentally hindered. In addition, refractive-index sensing of gas molecules using plasmons are also approaching very high sensitivity 18, 19, 20, 21. Recently, the sensitivity of electrical devices has been improved to the single-molecule level using nanomaterials 11, 12, 13, 14, 15, 16, 17. For example, for diagnostics, the presence of NO in the breath of patients is typically associated with chronic obstructive pulmonary disease 5, 6, while isopropanol 7, 8 and ammonia 9, 10 in the breath of patients are normally linked to lung cancer and renal failure disease, respectively. Label-free identification of gas molecules is very desirable for applications such as high-quality chip fabrication in semiconductor technology 1, detection of explosives 2, and medical diagnostics 3, 4. The demonstration and understanding of gas molecule identification using graphene plasmonic nanostructures open the door to various emerging applications, including in-breath diagnostics and monitoring of volatile organic compounds.
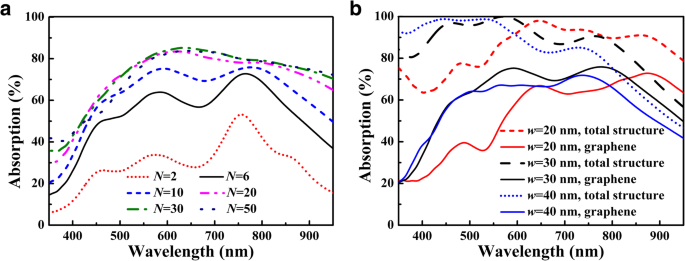
We further demonstrate a fast response time (<1 min) of our devices, which enables real-time monitoring of gaseous chemical reactions. The detected signal corresponds to a gas molecule layer adsorbed on the graphene surface with a concentration of 800 zeptomole per μm 2, which is made possible by the strong field confinement of graphene plasmons and high physisorption of gas molecules on the graphene nanoribbons. Here, we report label-free identification of gas molecules SO 2, NO 2, N 2O, and NO by detecting their rotational-vibrational modes using graphene plasmon. However, the most widely used gas nano-sensors are based on electrical approaches or refractive index sensing, which typically are unable to identify molecular species. Identification of gas molecules plays a key role a wide range of applications extending from healthcare to security.
